This article includes a list of general references, but it lacks sufficient corresponding inline citations. (May 2024) |
The retirement of a spacecraft refers to the discontinuation of a spacecraft from active service. This can involve deorbiting the spacecraft, discontinuing of the probes operations, passivating it, or loss of contact with it. One notable example of spacecraft retirement is Cassini's retirement in 2017.[1][2]
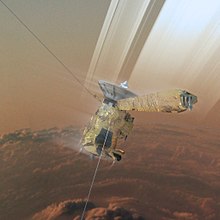
History
editThe first spacecraft to be retired was the Soviet Union’s Sputnik 1 probe. Launched in 1957, Sputnik 1 completed its mission when it naturally deorbited due to atmospheric drag after approximately three months in orbit, completing a total of 1,440 orbits around Earth.[3]
In 1958, NASA launched the Vanguard 1 probe as part of its efforts in the Space Race. Six years later, NASA officially concluded the mission as the probe had fulfilled all its experimental and objective requirements. Initially, NASA estimated that Vanguard 1 would remain in low Earth orbit for up to 2,000 years. However, due to solar radiation pressure and atmospheric drag, this estimate has been revised. Vanguard 1 is now expected to re-enter Earth’s atmosphere and burn up in approximately 240 years.[4][5]
Following the discontinuation of the Vanguard 1 probe, many space agencies and subsequent spacecraft have ceased operations through various methods.
In 2017, the Cassini–Huygens probe concluded its 19-year exploration mission by descending into Saturn’s atmosphere. The decision to end the mission was prompted by the minimal power supply remaining in the probe’s radioisotope thermoelectric generators (RTGs). The maneuver began on November 29, 2016, when Cassini performed a flyby of Saturn’s moon Titan, positioning it into the orbital plane of Saturn’s F Ring for its grand finale.[6][7] Cassini conducted another flyby of Titan on April 22, 2017, positioning the probe to pass within a precise 3,100 km (1,900 mi) of Saturn’s clouds. This maneuver was repeated 22 times until September 15, 2017, at 11:55:46 UTC, when communication with Cassini’s high-gain antenna was lost during the final flyby of Saturn. Cassini’s grand finale represented the first intentional entry of a spacecraft into the atmosphere of a gas giant and marked the first major spacecraft to burn up in such an atmosphere. The mission’s success has significantly influenced the planning and execution of both current and future missions focused on gas and ice giants.[8]
Reasons
editPower decrement
editMany modern spacecraft utilize radioisotope thermoelectric generators (RTGs), and spacecraft electric propulsion as their power source. Over time, the power output of RTGs or SEPs diminishes due to the gradual depletion of the radioactive isotopes they rely on for electricity generation.[9]
Radioisotope thermoelectric generators convert the heat generated by the decay of radioactive isotopes into electricity, providing a reliable power source for spacecraft equipped with RTGs. As the radioactive isotopes decay over time, the power output of the RTGs gradually decreases. It’s estimated that the power output from the RTGs will fall below the threshold required to operate the spacecraft’s systems effectively, including communication with Earth and scientific instruments. As a result, spacecraft equipped with RTGs will eventually run out of power sufficient enough to power their instruments.[10][11]
Spacecraft electric propulsion systems utilize electric fields to accelerate charged ions to high velocities, generating thrust for propulsion. Unlike chemical propulsion systems, which rely on the combustion of propellant, electric propulsion systems ionize propellant using electrical energy and then accelerate the resulting ions to produce thrust. As the ions are expelled from the spacecraft at high speeds, they create thrust in the opposite direction, enabling spacecraft maneuverability. Electric propulsion systems offer high efficiency and long-duration capabilities, making them suitable for precise trajectory adjustments, extended deep space missions, and potential future crewed missions. These systems rely on electrical energy for ionization and acceleration of propellant, typically supplied by onboard power sources such as solar panels or batteries. However, over time, these energy sources may become depleted, particularly in missions with extended durations or in environments with limited sunlight. Additionally, the components of SEP systems, including power electronics and ionization chambers, may degrade due to exposure to harsh space conditions, leading to decreased efficiency and power output. Furthermore, some SEP systems have a limited supply of propellant, such as xenon gas, which can be exhausted over the course of a mission, rendering the system unable to generate thrust. Ultimately, the end of a spacecraft's mission or operational life may also result in the cessation of power generation by the SEP system, marking the conclusion of its propulsion capabilities.[12][13]
Degradation of instruments
editSpacecraft instruments are susceptible to various external factors, including solar wind and other space phenomena. Solar wind, consisting of charged particles emitted by the Sun, can disrupt sensitive instruments like magnetometers and particle detectors, potentially reducing sensitivity or causing complete loss of functionality. Other space phenomena, such as cosmic rays and micrometeoroid impacts, also pose risks, potentially damaging spacecraft components and degrading instrument performance over time. Additionally, temperature extremes and outgassing of materials in space can further impact instrument functionality.
Loss of contact
editCommunication loss between spacecraft and Earth is a known occurrence, particularly as spacecraft travel deeper into space. Factors like distance, technical malfunctions, or power source depletion can all contribute to this loss. Efforts may be made to re-establish communication, but success isn’t guaranteed due to technological limitations or the spacecraft’s condition.
Pioneer 10 and Pioneer 11 serve as prominent examples of spacecraft encountering communication failures with Earth. As these probes traveled deeper into space, the distance between them and Earth grew, posing challenges for maintaining contact. Ultimately, their antennas dropped below Earth’s radio detection threshold, leading to the loss of communication.
Methods
editDeorbitation
editWhen spacecraft lose funding or complete their scientific objectives, they may alter their trajectories in various ways. One common approach is to redirect the spacecraft towards a path where it will burn up in the atmosphere of the celestial body they were orbiting. This intentional reentry minimizes the risk of the spacecraft becoming space debris, or helps the contamination of planets or moons.
Mission completion
editSome spacecraft are retired upon completing their scientific objectives. Depending on the mission’s requirements and circumstances, various retirement strategies may be employed. For example, spacecraft can be intentionally deorbited, Alternatively, they may be left in orbit around a planet or moon, however, leaving a spacecraft in orbit around a planet or moon carries the risk of contamination or the creation of space debris. Contamination could occur if the spacecraft carries microbes or organic material from Earth, potentially affecting the planetary or lunar environments. Additionally, if the spacecraft were to malfunction or fragment in orbit, it could contribute to the accumulation of space debris, posing hazards to other spacecraft and future missions. Therefore, decisions regarding the fate of retired spacecraft must carefully weigh these risks and implement measures to mitigate them.
Long-term orbital stability
editUnder certain circumstances, scientists can adjust a spacecraft's orbit to ensure it remains at a safe distance from the celestial body it's orbiting. This adjustment can prolong the spacecraft's orbital stability for hundreds of thousands to millions of years, mitigating the effects of planetary gravity, atmospheric drag, or solar radiation pressure. One notable example is NASA's Laser Geodynamics Satellite (LAGEOS), launched in May 1976. LAGEOS carries a time capsule, created by Carl Sagan, intended to burn up in Earth's atmosphere approximately 8.4 million years from now.[14]
See also
editReferences
edit- ^ Johnson, Nicholas (2011-12-05). Livingston, David (ed.). "Broadcast 1666 (Special Edition) - Topic: Space debris issues" (podcast). The Space Show. 1:03:05–1:06:20. Retrieved 2015-01-05.
- ^ Brown, Dwayne; Cantillo, Laurie; Dyches, Preston (September 15, 2017). "NASA's Cassini Spacecraft Ends Its Historic Exploration of Saturn". NASA. Retrieved September 15, 2017.
- ^ "Sputnik-1 1957-001B". NASA. 27 April 2021. Retrieved 16 May 2021. This article incorporates text from this source, which is in the public domain.
- ^ https://pages.vassar.edu/realarchaeology/2023/12/03/a-closer-look-at-the-archaeological-importance-of-the-vanguard-1/
- ^ Rosenthal, Alfred (January 1982). "A record of NASA space missions since 1958". NASA Technical Reports Server. NASA. hdl:2060/19940003358. Retrieved 24 September 2011.
- ^ "2016 Saturn Tour Highlights".
- ^ Lewin, Sarah. "Cassini Mission Kicks Off Finale at Saturn". Scientific American. Retrieved November 30, 2016.
- ^ Dyches, Preston; Brown, Dwayne; Cantillo, Laurie (April 27, 2017). "NASA Spacecraft Dives Between Saturn and Its Rings". NASA / Jet Propulsion Laboratory. Retrieved May 2, 2017.
- ^ Krauss, Lawrence M. (5 September 2017). "Pondering Voyagers' Interstellar Journeys, and Our Own". The New York Times. Retrieved 5 September 2017.
- ^ "The Actinide Research Quarterly: Summer 1997". lanl.gov.
- ^ Segal, Michael (1 September 2017). "Beyond Voyager". Nautilus. Archived from the original on 2 September 2017. Retrieved 2 September 2017.
- ^ "ESA Science & Technology - Electric Spacecraft Propulsion". sci.esa.int. Retrieved 27 April 2024.
- ^ Camilleri, Vincent (11 May 2017). "Electric Propulsion and Electric Satellites" (PDF). University of Colorado. Retrieved 27 April 2024.
- ^ Brabaw, Kasandra (25 May 2016). "NASA Put a Big Disco Ball in Space 40 Years Ago, and It's Still There". Space.com. Retrieved 3 May 2024.